2-D Or Not 2-D
8 Jan, 2007 01:34 pm
Over a century ago, when he invented the ubiquitous dish that bears his name, Julius Petri, a technician in Robert Koch?s laboratory, fundamentally transformed our ability to culture, manipulate and analyze cells. Since then, the Petri dish has become ubiquitous in biology laboratories around the world.
Geometric forms of 2-D and 3-D are drastically different in many ways. 2-D describes surface, but 3-D describes space. For example, land animals, dog, cat, caw, horse, tiger and human can only move on surfaces of plains, valleys and mountains; but birds and fish move in 3-D space, not only right and left but also up and down. Such an extra dimension gives them much more freedom than land animals. Cells in our body also move in 3-dimensions, from cells in our brain, to cells move to repair tissue damage to tumors move to seed the remote sites, or metastasis. However, cells have been mostly studied in 2-D or in poorly defined 3-D materials with unknown ingredients.
The architecture of the environment of a cell in a living organism is 3-D, cells are surrounded by other cells, where many extracellular ligands including many types of collagens, laminin, and other extracellular matrix proteins, not only allow attachments between cells and the basal membrane; but also allow access to oxygen, hormones, and nutrients; removal of waste products and other cell types associated in tissues. The normal 3-D environment of cells consists of a complex network of collagen matrix nanoscale fibers that create various local microenvironments, resembling numerous rooms in a sophisticated architectural building complex in a city as large as London, Tokyo, New York or Shanghai.
3-D nanofiber networks for neural stem cells
Neural stem cells grew, multiplied and morph into brain cells on a new nanofiber made scaffold of short designer peptides to be more like a living body than any other cell culture system.
The invention – made by Shuguang Zhang, associate director of MIT’s Center for Biomedical Engineering, and Fabrizio Gelain, a former MIT engineer now a researcher at the University of Milan - has been reported in the Dec. 27 issue of the Public Library of Science (PLoS) ONE.
Biomedical researchers have become increasingly aware of the limitations of growing living cells in coated two-dimensional Petri dishes and glass slides.
In the body, cells are indeed attached to and supported by the cells, other structures and proteins around them. A cell’s normal environment is a complex network of tiny fibers, gaps and pores through which oxygen, hormones and nutrients are delivered and waste products filtered away. Cells move within their natural environments in response to chemical signals or other stimuli.
The researchers were able to grow and to differentiate a healthy colony of adult mouse neural stem cells on the three-dimensional scaffolds that better represent the natural context of cells in tissues and organs without the drawbacks of two-dimensional systems.
Researchers are aware that cells on flat surfaces have skewed metabolisms, gene expression and growing patterns. But the only choices have been glass labware and a product called Matrigel, a gelatinous protein mixture secreted by mouse tumor cells. While Matrigel does resemble a complex extracellular environment, it also contains growth factors and unknown proteins that limit its desirability for experiments requiring precise conditions.
Shuguang Zhang is a pioneer in coaxing tiny fragments of amino acids called self-assembling peptides to organize themselves into useful structures. Working with visiting graduate student Fabrizio Gelain from Milan, Zhang created a designer scaffold from a network of protein nanofibers, each 5,000 times thinner than a human hair and containing pores up to 20,000 times smaller than the eye of a needle.
In addition to helping researchers get a more accurate picture of how cells grow and behave in the body, the new synthetic structure can provide a more conducive microenvironment for tissue cell cultures and tissues used in regenerative medicine, such as skin grafts or neurons to replace brain cells lost to injury or disease.
The scaffold itself can be transplanted directly into the body with no ill effects. Another MIT researcher has used the same peptides in a biodegradable liquid that can stop bleeding within seconds and restore the function of damaged optical nerves. The protein fragments form a miniscule protective barrier. Once the injury heals, the nontoxic scaffold is broken down into molecules that cells can use as building blocks for tissue repair.
With the addition of defined amino acid fragments (active and functional motifs), the scaffold can be tail-made to coax cells to behave in certain desirable ways—such as proliferating or differentiating into needed body tissues.
What makes these designer scaffolds particularly interesting is that cells survive longer and differentiate better without additional soluble growth factors. This suggests that extracellular microenvironments may play a more important role for cell survival and for carrying out cell functions than previously thought.
The active motif method could be readily adapted to studying cell-to-cell interaction, cell migrations, tumor and cancer cell interaction with normal cells, cell-based drug testing, gene involved in cell adhesion and differentiation.
The researchers are now testing the designer scaffold with a variety of cells, including tooth, bone, heart, liver, cartilage, skin, pancreas, blood cells and artery-forming cells. They are also testing the scaffolds for tissue engineering applications like peripheral nerve regeneration and spinal cord injury.
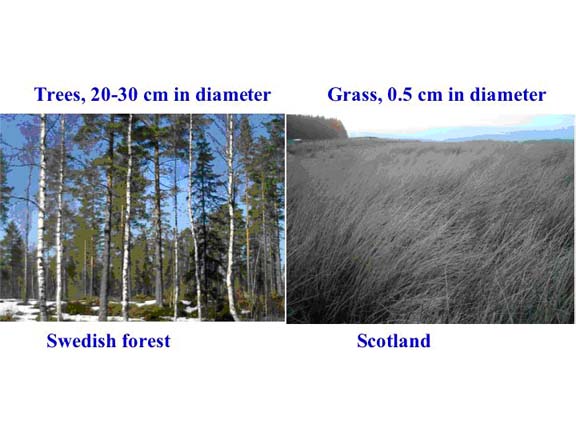
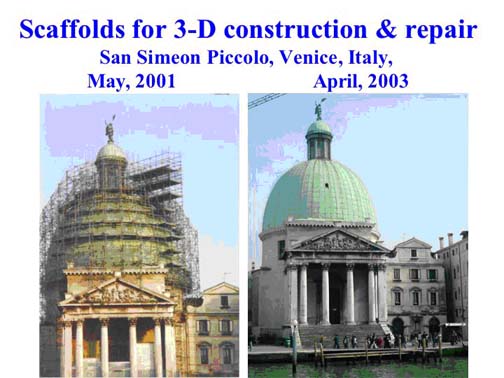
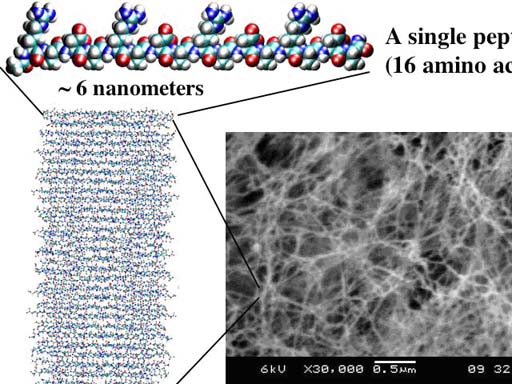
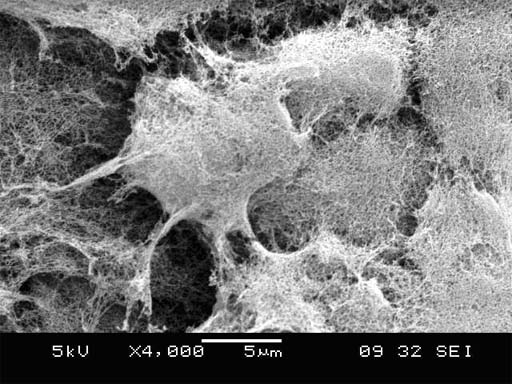
Fig. 4. Cells are fully and
intimately embedded in 3-D self-assembling peptide nanofiber scaffold. Cells are ~10 micron in size, and the
nanofibers are 10-nanometer in thickness. The cells are embedded in the scaffold, similar as the scaffolds embed
the San Simeon Piccolo Dome in
Article by Dr. Shugang Zhang and Dr. Fabrizio Gelain.
1 comment(s)
[1]
Comment by Ahmad
16 Apr, 2007 01:23 pm
i have a quation how cam i design a scaffold?
