Key words :
nanoscience,
nanotechnology
,nanoscience
,diffraction limit
,gold nanorods
,optical antenna
,imaging
Quantum Cascade Laser Nanoantenna
21 Nov, 2007 10:54 am
Imaging techniques with high spatial resolution in the mid-infrared region of the optical spectrum (wavelength ranging from 3 microns to 25 microns) have always been attractive and desirable because the characteristic absorption peaks of most chemicals, known as ?fingerprints? , reside in that region. However, conventional imaging or spectroscopy techniques have a limited resolution on the order of ten microns.
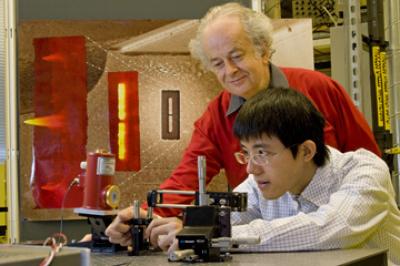
A quantum cascade laser nanoantenna is designed to break this “diffraction limit” in the mid-infrared region. By defining an optical antenna on the output facet of a mid-infrared laser source (quantum cascade laser), a compact device of no more than a couple millimeters in length and several tens of microns in width can be constructed that is capable of performing imaging with a spatial resolution on the order of 10 to 100 nm (about 1/1000 to 1/100 of a mid-infrared wavelength).
What is an optical antenna and how does it work? The optical antenna is composed of a pair of gold nanorods separated by a nanometers size gap between them. In the image shown here, the gap size is 100 nm and the laser had a wavelength of 7 microns. The antenna is able to collect incident light and generate an electric current flow inside the antenna: much like the antenna hooked to your radio, which captures the radio wave in the air and converts it to an electric current signal. The current generated in the optical antenna produces a large accumulation of electric charges at the antenna ends that define the antenna gap, thus leading to a localized, strong optical field in the antenna gap. The advantage of an optical antenna is that it can capture the incident light of an area much larger than the geometric area of the thin antenna rod itself. This effectiveness in collecting radiation is strongest when the length of one antenna rod equals half of the incident wavelength. So effectively, an optical antenna of the “right size” behaves like an optical “funnel”: it efficiently collects the energy of the laser output and concentrates it into the tiny area of the antenna gap. The size of the resulting tiny optical spot is largely determined by the size of the gap, rather than the wavelength of the laser. As is illustrated below, the size of the optical spot basically determines the imaging resolution.
Now imagine that we are performing a raster-scan of a specimen, this time using the tiny optical spot produced by the quantum cascade laser nanoantenna. When the optical spot is on a part of the specimen that does not absorb the mid-infrared light in use, there would be a strongly scattered optical field originated from the interaction of the optical spot and the specimen. On the contrary, in a region where the sample is highly absorbing, there would be little such scattered light. Thus, by collecting the power of the scattered light as the spot is scanned across the sample, one can get a chemical map, from which materials with different absorption “fingerprints” can be clearly distinguished. The resolution of the map is determined by the size of the optical spot and the scanning step, not by the wavelength of the mid-infrared source in use. The merit of a quantum cascade laser is that one can custom-design its lasing wavelength, covering the entire mid-infrared spectrum. Therefore, in order to recognize one specific chemical in a specimen, we can tune the lasing wavelength of the quantum cascade laser to match one of the characteristic absorption peaks of the chemical. Of course, the optical antenna defined on the laser facet also needs to be tuned to the “right size” in order to match the wavelength of the laser.
In summary, the quantum cascade laser nanoantenna may find important applications in high spatial resolution chemical and biological imaging and spectroscopy.
Reference
1. Nanfang Yu, Ertugrul Cubukcu, Laurent Diehl, Mikhail A. Belkin, Kenneth B. Crozier, Federico Capasso, David Bour, Scott Corzine, and Gloria Höfler. Plasmonic quantum cascade laser antenna. Applied Physical Letters 91, 22 October 2007.
Key words :