Transferring Quantum Information from Light to Matter and Back Again
12 Jun, 2007 02:18 pm
A quantum computer is a device that can perform certain tasks much more efficiently than a classical computer byexploiting unique aspects of quantum mechanics, such as quantum coherence and entanglement. Such a device has yet to be built, however over the past decade experimental research in the field of quantum information processing has made many promising advances, including the work described in our recent publication [1] on reversible state transfer between light and matter.
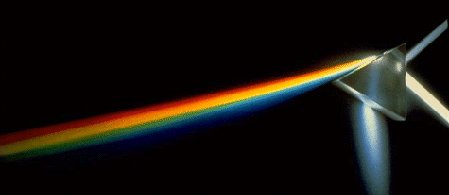
the next.
Within the nodes of the network, where the quantum information is stored and acted-upon via logic gates, the qubits are stationary. Therefore, they can be encoded in the internal states of single atoms, which are particularly long-lived and relatively easy to manipulate. However, for the purpose of conveying quantum information from one node to another, the qubits need to be in a form that is easy to shuttle around. Therefore, a good way to encode such "flying" qubits is in the number of photons or in the polarization states of light, which is easily transported in either free space or optical fibers. In addition, there needs to be a way to turn stationary qubits into flying qubits and vice-versa, and to do that in a coherent, reversible fashion. That is where cavity quantum electrodynamics (QED) comes in, since it provides a way to coherently map atomic states onto states of light and back.
Cavity QED is a branch of physics dealing with the interaction of a single atom with a single mode of electromagnetic radiation, such as a well-defined wavelength of light. In our cavity QED laboratory at Caltech we have a tiny optical cavity formed by two extremely high-quality curved mirrors, which are about 40 microns apart. The mirrors are so reflective that a photon inside the cavity will bounce back and forth about 450,000 times between the two mirrors before it escapes. A single Cesium atom is confined within the cavity by an optical "tweezer", and laser-cooled to a temperature within a small fraction of a degree of absolute zero. The resulting parameters for this atom-cavity system are such that quantum information can be exchanged many times between the atom and the light field before the coherence is lost. This parameter regime is known as "strong coupling".
In our most recent experiment [1], the atom undergoes a series of light absorption and reemission cycles. During each such cycle, the cavity is first illuminated by an incident pulse of laser light, whose state represents the input, which is a flying qubit. Whenever the atom-cavity system absorbs this pulse, its state is written onto the internal state of the atom, which is a stationary qubit. After a fixed delay, we map the atomic state back onto an emitted pulse of light, which is the output qubit. This output pulse is then allowed to interfere with the source of the original, input pulse. The result that we measure is an interference fringe (conceptually analogous to the fringe in Young's double slit experiment), which proves that mapping the qubit from light to atom and back to light preserves its coherence. Thus, an important ingredient for a quantum network, namely the ability to turn flying qubits into stationary qubits and vice-versa in a cavity QED setting, is demonstrated in the laboratory for the first time.
Reference:
[1] A. D. Boozer, et al. Reversible State Transfer between Light and a Single Trapped Atom Physical Review Letters 98, 193601 (2007).